30 The Aging Process Explained
Sabine Zempleni and Sydney Christensen
When you think about your parents, relatives, friends, and acquaintances, you will notice that you know some very healthy and fit 60-year-old people and 60-year-old people that have trouble functioning in daily life.
Naturally, scientists are interested in finding out why some people age faster than others. One type of study that allows us to study aging is twin studies. In this type of study identical twins are recruited and studied. Since identical twins have identical DNA any differences between those identical twins can be assumed to be due to environmental factors.
Twins that showed the greatest discrepancies in aging also had the greatest degree of discordance between personal lifestyle choices and habits. The most notable exogenous factors that influenced the degree of aging were sun exposure and smoking. Other contributors included excessive alcohol consumption, stress, diet, exercise, disease, and medications.
Twin studies indicate that the genetic influence on aging may be overrated, with lifestyle choices exerting important effects on physical aging. Once an unhealthy lifestyle results in obesity and chronic diseases, the aging process becomes even more accelerated.
Although we know what factors are linked to increased aging, we are just beginning to understand the metabolic processes that accelerate aging. The hope is, once we understand the metabolic processes of aging, we can find ways to slow the aging process down.
Here is an overview of what we know today.
You Will Learn:
1. Physiological aging is ongoing through adulthood
- Decline of bone density starts during the third decade of life.
- Maintaining body composition during adulthood is hard work.
- Menopause equals the playing field for men and women.
- Subtle endocrine decline takes place in healthy adults but is not well researched.
2. Going Deeper: Cellular aging affects virtually every aspect of cell biology
- Mitochondria dysfunction reduces the production of ATP increasing fatigue and impacting organ function.
- ER stress leads to cellular garbage triggering the innate immune system and fueling inflammaging.
- DNA damage and changes to the epigenome increase the risk for age-related chronic diseases.
3. It is estimated that genes contribute 15-30% to the aging process, while lifestyle choice can speed up or slow down aging.
Physiological Aging Is Ongoing Through Adulthood
Review Some Basic Osteoporosis Facts First
Before you learn about the declining bone density during adulthood, you should first review a few facts about osteoporosis, bone remodeling, and the interaction between calcium and vitamin D:
Osteoporosis is a common medical condition especially in older women. Around a quarter of American women over 65 and around a third of women over 80 will develop osteoporosis. For men the prevalence is lower. Looking at those prevalences it becomes clear that women are more affected by osteoporosis than men and that osteoporosis prevalence increases with age. When we look at health disparities Americans with hispanic origin are most affected, followed by white Americans. Black Americans have the lowest prevalence.
Osteoporosis is diagnosed when bone density is so low that bones become brittle. Loss in height and spontaneous fractures occure. Before osteoporosis is diagnosed affected people go first through low bone mass called osteopenia. Since low bone mass is a risk factor for osteoporosis a bone mineral density test (DEXA scan) is performed after age 65, earlier if the individual has a high risk for osteoporosis.
Risk factors for osteoporosis can be categorized into two groups, modifiable and non-modifiable.
The first non-modifiable risk factor is age since bone mass naturally declines with age. Second, a genetically low peak bone mass at age 20. Anybody who is small boned such as women, especially Asian, white and Hispanic women, has genetically an increased risk to develop osteoporosis. Smaller bones have a smaller mineral reservoir. Once bone mineral loss starts after age 30, there is simply not as much to lose. Lastly, going through menopause early will accelerate bone loss.
On the modifiable side the main factor is again a low peak bone mass at age 20, this time due to life style. Adequate intake of calcium and vitamin D are essential to reach the maximal bone mass. Low BMI, smoking and excessive alcohol consuption also impact bone mineral density.
Bone (mineral) density is the amount of minerals—mostly calcium but also phosphorus, magnesium and potassium—deposited in the lattice like protein structure of bones. It is important to understand that bones are constantly rebuild. This process is called bone remodeling. Two cell types located on the bone surface are involved in this process. Osteoclasts are continuously dissolving bone while osteoblasts are building bones. Inside the bone is a third cell type, osteocytes. Osteocytes remove and add minerals to the bone structure.
Reason for this constant remodeling is the function of the bone as a storage site for calcium and phosphorus. Note that mineral storage has priority over strong bones. Osteocytes release the minerals into the blood stream when blood levels are low.
An adequate calcium and vitamin D intake will ensure that the bones are properly mineralized in childhood and that not too much bone minerals are lost during adulthood. The best source for calcium is milk, dairy products and calcium fortified plant milks. Some plants can have good amounts of calcium but bioavailability tends to be low.
Adequate amounts of vitamin D are necessary to absorb the calcium. During the summer our body produces enough vitamin D if we get sufficient sun exposure without sunscreen. The lack of sunscreen is not problematic here because exposure of hands, arms and face for 10 – 30 minutes (depending on skin color) two to three times a week is sufficient. Of course, if somebody plans on spending more time in the sun or during UV peak hours at noon, sunscreen is a necessity to prevent skin cancer. Living in Northern latitudes in winter or spending most of the time indoors makes it necessary to eat vitamin D. Vitamin D can be found in fatty fish, egg yolk and fortified foods such as vitamin D milk. A Vitamin D supplement might become necessary.
Decline of Bone Density Starts During the Third Decade of Life
When you read in the media about dairy and bone strength, the science seems chaotic. Claims range from dairy prevents osteoporosis to dairy causes osteoporosis, all of course supported by “science”. To understand those seemingly contradictory statements, you need to understand how bone mineral density develops over a lifetime.
Infants are born with very soft and malleable bones. This allows the fetus to squish through the narrow birth canal. Right after birth, the deposition of minerals into the collagen structure of the bone begins and will last all through childhood and adolescence.
During adolescence, bone mineralization accelerates until peak bone mass—the highest level of bone mineralization a person can ever reach—is achieved. During this phase of life, bone-building osteoblast activity outpaces bone-dissolving osteoclast activity. The result is an increase in bone density.
After peak bone mass is reached, bone mineralization plateaus for a short time (osteoblast activity = osteoclast activity) and then starts to decline very slowly until age 50 is reached. During this phase of slow bone loss, the bones are completely remodeled every 10 years, but bone dissolving osteoclast activity is now slightly higher than osteoblast activity. After age 50, the loss of bone mass accelerates until the end of life.
Therefore, the main factor in determining if somebody will develop osteoporosis over a lifetime is the peak bone mass. The higher the peak bone mass of a young adult, the less likely it is that the person develops osteoporosis later in life. Genetics control 80% of the variation in peak bone mass between individuals. For instance, small-boned individuals start out with less minerals to lose over a lifetime. This is one of the reasons why women, especially Asian women, have a higher risk for osteoporosis.
Optimal Calcium And Vitamin D Intake Combined With Exercise Ensure a High Peak Bone Mass And Slow Bone Loss
The genetically predetermined peak bone mass can only be reached with an adequate calcium and vitamin D intake, along with sufficient exercise.
To understand how those three factors work together, we need to realize that the bone is a storage site, a reservoir for calcium and other minerals. 99% of all calcium in the body and 85% of phosphorus is stored in the bone.
Calcium blood concentrations must be maintained within a narrow range to enable muscle contraction, nerve function and intracellular processes. If calcium intake is low and blood calcium levels fall, parathyroid hormone (PTH) acts on the osteoclasts, and calcium is released from the bone. If release of calcium from the bone storage site is ongoing the bone loses strength.
This means that there are two separate concepts when it comes to calcium and bone strength:
- Children and adolescents must have an adequate intake of calcium to reach the highest peak bone mass possible which is best accomplished by eating dairy or dairy replacements (see calcium source table).
- Adequate intake of calcium during the rest of the life will ensure that bone mass loss is as slow as possible. However, increased calcium intake will not replace bone mass that is already lost or was never reached during the first 20 years of life.
What Is an Excellent Calcium Source?
It is important to keep calcium bioavailability and usual servings sizes in mind when judging food sources. The table shows how much calcium is in a cup of the food, the bioavailability, and how much food would be necessary to replace the calcium content of 1 cup of milk (remember daily recommendation is 3 cups per day) with another food source.
When we look at calcium content in foods, we see that aside from milk and dairy, some leafy greens look promising as calcium sources.
Although many leafy greens contain good amounts of calcium, they also contain oxalic acid. Oxalic acid binds calcium and makes it unavailable for absorption. It becomes clear that, while vegetables contribute to calcium intake, it is very difficult to get the recommended amount of calcium from vegetables alone. Who can eat 12 cups white beans or 9 cups kale each day? If dairy consumption is not possible due to lactose-intolerance, fortified foods need to be considered.
Adequate calcium intake alone is not sufficient though, as vitamin D deficiency contributes to bone loss. Calcitriol, the active hormone form of vitamin D, acts on the small intestine to increase calcium absorption from food when blood calcium levels fall. If circulating vitamin D blood levels are inadequate, calcitriol cannot be synthesized, and calcium will not be absorbed. The missing calcium in the blood will need to come from bones.
In addition to adequate calcium and vitamin D intake, weight bearing exercises, such as strength training and higher impact aerobic activities, stress the bone. This triggers repairs, and therefore better bone mineralization of the bone in all life stages.
In summary, genetics determine the maximal peak bone mass a person can reach; however, intake of calcium and vitamin D as well as exercise determine if the maximal peak bone mass is reached and how fast the bone mass is lost during life.
Two Phases of Life Are Especially Important to Maintain as Much Bone Mass as Long as Possible: Adolescence and Menopause
Adolescence: Infants and young children in the US drink sufficient amounts of milk. However, during teenage years—when bone mineralization accelerates—milk and dairy consumption tends to fall short. As a result, many teens do not get enough calcium to reach their genetically maximal peak bone mass. Another contributor to this issue is a sedentary lifestyle. Children and teens should be encouraged to be active and exercise so they can reach optimal peak bone mass.
Menopause: During menopause the cessation of estrogen production stimulates osteoclast—bone dissolving—activity. Consequently, women will go through a phase of accelerated bone mass loss for a few years after menopause. Then bone loss slows down again (see graph on the ride side of the infographic above). This short period of rapid bone mineral loss partially explains the higher risk for osteoporosis in women compared to men.
Underweight young women and women with eating disorders will experience a decline of estrogen blood levels due to declining leptin levels. These women also show signs of accelerated bone loss independent of age. Once those underweight women gain weight, estrogen production resumes and bone mineralization normalizes. The bone loss or reduced bone mineralization cannot be reversed though and will increase the risk of developing osteoporosis later in life.
Lastly, inadequate calcium and vitamin D intake during pregnancy will also lead to accelerated bone loss that cannot be reversed after pregnancy. During the pregnancy the women’s body prioritizes the fetus. Low calcium blood concentrations are balanced by releasing calcium from the bone.
Ideally, Osteopenia Will Happen in High Age but Osteoporosis Never Will
Bone mineral density and osteoporosis are not conditions adolescents and younger adults think about. All individuals will, at one point during their lifespan, lose enough bone mass to develop low bone density, called osteopenia. It is just a question of longevity. The goal is to reach this point as late as genetically possible, and that osteoporosis with brittle bones and spontaneous fractures is never reached.
Low bone mass is diagnosed using a DEXA scan. Many women undergo a DEXA scan during or after menopause as a preventative measure to detect osteopenia. The general recommendation for both men and women is to undergo a DEXA scan after 65.
Low bone mass should be an alarm bell because osteopenia—if bone loss keeps progressing—will develop into osteoporosis along with an increased risk for fractures and loss of life quality as seen in the video clip below.
The diagnosis of osteopenia or osteoporosis should trigger lifestyle changes such as better calcium and vitamin D intake and increased exercise. Bone loss cannot be reversed with lifestyle changes, but further bone loss can be slowed down. Lately, bone loss reversing medications are available, and can help gain some bone mass back and avoid further loss.
Keep in mind that this situation can be easily prevented during childhood and adolescence.
Coming back to the seemingly confusing science, studies are clear that a good calcium intake from dairy or dairy replacements is necessary to reach genetically optimal peak bone mass during childhood and adolescence. Milk and many of the plant milks are also enriched with vitamin D. Exercise and outdoor activities complement the calcium intake. This needs to be separated from studies conducted in middle aged adults and seniors since for this group the goal is slowing down bone loss. Based on the physiology, increasing dairy intake cannot be expected to increase bone mass in later life.
Maintaining Body Composition During Adulthood Is Hard Work
Terminology:
Fat mass and fat-free mass are expressed as percentages of body weight. Fat-free mass is determined by subtracting the fat mass, determined by a traditional method to determine the body fat content, from the body weight. Keep in mind that this means that if fat-mass goes up, fat-free mass must go down and therefore you will be only able to judge the relationship between FM and FFM. DEXA can determine lean body mass (LBM) and is therefore more precise. Determining LBM will allow us to know how much muscle a person has compared to fat mass.
Fat-free mass (FFM) includes water, electrolytes, organs, bones, muscles, and blood. Fat-free mass is, on average, 72.5% water and 20.5% protein and minerals. Keep in mind that this is an oversimplification because water content varies between tissues. For example, bone has a water content around 22% and the brain 75%.
FFM and lean body mass (LBM) are often used interchangeably, sometimes even in textbooks, but this is not correct:
- FFM = Body weight – Fat Mass
- LBM = Body weight – Fat Mass – Mineral Deposits – Extracellular Fluids
Fat Mass (FM) is about 79% fat, 3% protein, and 18% water. Fat mass or adipose tissue is found under the skin (subcutaneous), within the body cavity (visceral), or within muscle and organs (ectopic).
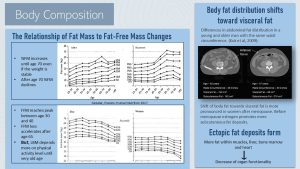
Fat Mass Increases with Aging Even if Weight Is Maintained
Body fat (%FM) increases slowly during aging. Interestingly, scans show this increase occurs even if weight is stable. This trend lasts until senior age, then body fat starts to decline around age 70. It is not clear what triggers this development, but we know genetic differences are involved because studies show that Asian adults seem to reach this peak in body fat earlier during their 50s.
In Middle Age Body Fat Redistributes from Peripheral to Central Fat Stores, From Subcutaneous to Visceral
As fat mass slowly increases during middle age, body fat redistributes from peripheral to central fat stores. There is also a shift from subcutaneous to visceral fat stores. As a result of these adjustments, waist circumference keeps increasing.
Before menopause, many women have a much lower amount of central adipose tissue compared to men; however, after menopause, waist circumference increases in women at the same rate as men. This is thought to be due to the loss of estrogen, which promotes a gynoid fat distribution (peripheral, subcutaneous) before menopause.
The image on the right of the infographic above compares a scan of the abdominal body fat distribution of an 82- and 37-year-old person. Both individuals have the same waist circumference. In this scan, muscles and organs are light gray while adipose tissue is dark gray. The 82-year-old (left) has less subcutaneous fat and more visceral fat (Kuk et al., 2009).
Ectopic Fat Deposits Form
Fat-Free Mass Declines During Adulthood
Men and women reach a peak of fat-free mass sometime between their mid 30s and 40s. The decline can start much earlier or later depending on the amount of exercise and physical activity. After age 65, this decline starts to accelerate.
We need to be cautious when interpreting the decline in FFM. Most traditional measurement techniques for body composition determine the fat mass. The FFM is determined by subtracting fat mass from body weight; thus, when body fat increases, FFM needs to decline. The more important question is whether we are also seeing a decline in LBM, which can be measured using DEXA. Lean body mass seems to be stable in physically active young and middle-aged individuals,. Loss with age occurs in sedentary and very old individuals.
Menopause Equals the Playing Field for Men and Women
Menopause Marks the End of Fertility for Women and the Start of a Whole New Life
Before menopause, estrogen and progesterone levels rise and diminish in a distinct cyclical pattern, roughly every 28 days.
Estrogen increases during the first half of the menstrual cycle, growing the uterus lining and stimulating the maturation of an ovum in the ovary. When estrogen reaches its peak, ovulation takes place and progesterone production increases. If the ovum is not fertilized, both estrogen and progesterone production declines. These declining hormone levels trigger the shedding of uterus lining, the menstruation. After the menstruation, estrogen secretion increases, and the menstrual cycle starts over.
Starting in the 4th decade of life—though the start varies widely—women enter perimenopause. Perimenopause lasts several years and is marked by a less regular cyclical estrogen and progesterone pattern and lower hormone levels. The consequence is infrequent periods and reduced fertility. Because estrogen is involved in body temperature regulation, many women will also experience the famous hot flashes, which may lead to sleep problems. The intensity of these symptoms varies from woman to woman.
Perimenopause concludes with menopause. Menopause is defined as the point in life when ovulation stops completely. Women are considered menopausal if they do not have a period for more than a year.
Estrogen Has Many More Functions in Addition to Fertility
We usually associate estrogen with female fertility, but estrogen has other important regulatory roles. Once estrogen blood levels decline during perimenopause, physiological changes set in.
We already talked about estrogen and bone density maintenance. Estrogen is bone-protective. Declining estrogen blood levels stimulate bone-absorbing osteoclast activity and accelerate bone loss for several years after menopause. This is a non-modifiable physiological process. Once the woman is a few years past menopause, bone loss slows down to a steady decline—similar to the rate for men.
The lack of estrogen also has metabolic consequences. Estrogen tends to be cardioprotective. One average, pre-menopausal women have lower LDL cholesterol—and thus have a lower risk for CVD and heart attacks—compared to men. After menopause, women “catch up” to men, and are at the same risk level.
High blood levels of estrogen promote a pear-shaped body type. After menopause, women experience two changes:
- It is much harder for women to maintain a healthy body weight
- Body fat distribution shifts towards central obesity
These changes, higher weight and central obesity, increase the risk for chronic diseases.
Myth or Fact: Do Women Experience Cognitive Decline During Menopause?
No, this is definitively a myth!
Studies show a slight cognitive decline during menopause—but this is mostly due to other factors such as increasing BMI, the aging process, or other health issues—and not specific to women.
On a side note, there is one exception. After having a full hysterectomy, the surgical removal of the uterus and ovaries, women undergo induced menopause because the ovaries—normally producing estrogen and progesterone—are missing. If this happens early in life, for example when women are in their 20s and early 30s, studies show that those women are affected by some degree of cognitive decline. The earlier the artificially induced menopause takes place, the more noticeable the cognitive decline is.
Maybe the results from those studies led to the overall notion that menopause and cognitive decline are connected; however, a cognitive decline is not observed if women are undergoing a timely natural menopause in their late 40s and early 50s.
Is there such a thing as andropause? The menopause for men?
No, andropause is a myth! However, men do undergo a moderate gradual decline in testosterone secretion from the peak in the early 20s until age 40. This natural decline in middle age—and a potential further decline throughout later life—is highly variable between individual men. Declining testosterone increases the risk for weight gain and muscle mass loss.
Subtle Endocrine Decline Takes Place in Healthy Adults, but Is Not Well Researched
Growth Factor and IGF-1 decline
Growth hormone and IgF-1, both necessary for growth and organ maintenance, reach a peak around 20 years of age. After reaching a peak, both steadily decline during adulthood; however, the consequences of this decline are not clear.
Decreasing growth hormone is discussed as a potential reason for a decline in lean body mass.
On the other hand, high IgF-1 levels are not desirable and are considered a risk factor for some cancers. Centenarians tend to have very low IgF-1 blood levels and scientists wonder if this is protective against cancer and other chronic diseases.
Feasting and high-protein diets increase IgF-1 blood levels. This is what led to wild media claims such as “high protein diets increase the risk for cancer” and “regular fasting results in longevity.” These connections were only observed in animal research, while in humans a slightly higher weight in high age seems to be more beneficial. More about that later.
It will be interesting to see where this research goes, but at this point, it is too early to start fasting for longevity or eating a low-protein diet to reduce IgF-1.
Beta-Cell Function Declines
You already learned about the decline of beta-cells in people with insulin resistance due to an unhealthy lifestyle and obesity.
Even in healthy active adults, the function of insulin-producing beta-cells in the pancreas declines 1% per year. In healthy people this decline is very subtle.
Since research primarily focuses on pathological insulin resistance and T2D, research into the physiological mechanisms in healthy adults is slim. It is thought though that this physiological decline in beta-cell function with age is not due to increased cell death, as is the case with insulin resistance and T2D. Instead, the functional decline seems to be due to reduced cell proliferation (cell division and growth). This is not unique to beta-cells; the regenerative capacity of cells decreases with age for most organs.
Due to declining beta-cell function, the risk of developing T2D increases with age in healthy adults. This age-effect is relatively small compared to the effect of weight and a sedentary lifestyle. Decreasing insulin sensitivity is much more connected to an increasing BMI, or more precisely, to an increasing waist circumference.
What about the “leaky” gut?
Going Deeper: Cellular Aging Affects Virtually Every Aspect of Cell Biology
There are various physiological changes we can evaluate when assessing aging: Determining body composition, drawing blood to measure an array of metabolic markers, and measuring hormone blood levels. When we do this, we see that everything becomes less efficient with age.
Part of this decline can be explained by an increase of ectopic fat in organs and the shift of fat deposits to central obesity. However, this does not explain the subtle decline of insulin production, the speeding up of atherosclerosis, or the decline of cognition during the senior years. It also does not explain why individuals with chronic diseases tend to age prematurely. For that, we need to dig deeper to the cellular level.
You may have read that eating a certain diet or taking a range of supplements might slow down aging. All those claims tend to center around antioxidants. Garlic and vitamin E were the first and most popular anti-aging supplements, until it was discovered that high doses of vitamin E can lead to brain bleeds.
Today, turmeric, EGCG from green tea, anthocyanins from red wine, or flavonols from dark chocolate are touted as the life-prolongers. What they all have in common is that they are anti-inflammatory. This is where a kernel of truth lies: When the aging process is examined at the cellular level, inflammation—measured, for example, by cytokine blood levels—increases with age.
Inflammaging: Cellular Aging Increases Inflammation
Research in animal models and human cell studies has demonstrated that aging affects all aspects of cell biology, likely due to the physical and chemical damage that happens throughout a lifetime.
The sources for this cell damage are either endogenous (for example, due to free radical production during cell metabolism) or exogenous (for example, due to external sources such as overnutrition, sun exposure, smoking, and excessive alcohol consumption). The constant attempts to repair this ongoing damage to cells and DNA exhaust the molecular mechanisms over time, and eventually errors are made. It is like a car you drive for 10 years; the components of the motor will experience wear and tear. Material weakens, and at one point breaks down.
When we want to understand how metabolically unhealthy obesity and chronic diseases accelerate aging we should look into two cellular aging processes: Mitochondrial dysfunction and the production of cellular garbage.
Mitochondrial Dysfunction: During normal energy metabolism ATP is produced in the electron transport chain. In addition to ATP production a by-product called reactive oxidant species, ROS for short, are produced as well. ROS are strong oxidants and grab electrons from fatty acids in the mitochondrial membranes triggering an oxidative chain reaction.
Where exactly are ROS produced?
Mitochondria have two membranes, a smooth outer membrane and a folded inner membrane. The inner mitochondrial membrane is the location of the electron transport chain, which is essential to the production of ATP. The electron transport chain functions by separating hydrogen donated by NADH+H+ and FADH2, produced in the citric acid cycle and beta-oxidation, into protons and electrons. The protons (H+) are transported across the inner cell membrane into the intermembrane space. The electrons are handed along protein carriers sitting in the inner cell membrane. This builds up an electrochemical gradient. The energy of this gradient is used to drive the oxidative phosphorylation of ADP to ATP. If everything goes to plan the electrons and protons are transferred to oxygen forming water.
As everything in nature, this transfer does not always go to plan and too many or not enough electrons and protons are transferred to water. Lack of electrons in the outer shell of an atom will make the atom very reactive. The molecule will now aggressively steal electrons from other molecules and in the process damage the molecules in the mitochondrion membrane.
Damage to the inner mitochondrial membrane leads to alterations in the function of the electron transport chain. Damage to other mitochondria membranes leads to a reduction in transport of critical metabolites, such as fatty acids, pyruvate, NADH+H+ into mitochondria.
Consequently, less ATP is produced, and the person with mitochondrial dysfunction will experience more and faster fatigue. A person with mitochondrial dysfunction, also called mitochondrial disease, will experience for example muscle weakness, excessive fatigue and cognitive decline. The risk for CVD and T2D increases. In healthy individuals mitochondrial dysfunction starts to develop in high age. This is the reason why many seniors report that they can complete everyday tasks but need to take short breaks to finish a task.
Increased ROS production also damages other cell organelles and the DNA. Damage to the cell increases inflammation.
Increased Cellular Garbage: After amino acid chains are produced at the ribosome using messenger RNA, the amino acid chains need to be folded properly to become functioning proteins. The endoplasmic reticulum (ER) is a cell organelle involved in the folding of proteins. Just like the mitochondria, ROS production affects the ER over time. This is called ER stress.
Damage to the walls of the ER results in a reduced capacity to fold proteins. Increasing amounts of misfolded proteins aggregate in the cell because the garbage disposal system of the cell, autophagy, becomes also less efficient during the aging process. This accumulation of misfolded proteins called cellular garbage—this is the actual scientific terminology—triggers an inflammatory response, which increases chronic inflammation.
DNA Damage: The DNA has now experienced 60-80 years of damage and repair. Some of those repairs were not successful. Mistakes while copying the DNA or the epigenome are carried forward to the daughter cells during further cell divisions.
Copy mistakes lead to gene mutations, and these in turn can produce neoplastic cells, cells with abnormal growth. Most neoplasms are detected by the immune system and removed. As cells age, more abnormal cells form which also increases inflammatory processes. The aging immune system allows more neoplastic cells to escape and grow into benign or malignant tumors. The risk for cancer increases.
Telomere Attrition: Independent from oxidative damage, telomeres—the protective caps at the tip of chromosomes—wear down gradually with every cell division. This is called telomere attrition. Over time, the telomeres become shorter and shorter, until the chromosomes cannot divide anymore. This will lead to apoptosis (cell death) which again will trigger an inflammatory response.
Damaged organelles, misfolded proteins, impaired disposal of cellular garbage, and increasing amounts of neoplastic and dying cells all trigger the innate immune system. Recall that the innate immune system is always triggered if a molecule is detected that should not be there. The age-related systemic inflammation is called inflammaging can be determined by measuring the increased proinflammatory cytokines in the blood.
Glucotoxicity and Lipotoxicity Accelerate Cellular Aging
In module one, you learned that metabolic syndrome, insulin resistance, T2D, and CVD often come with elevated free fatty acid and glucose blood concentrations. Both are toxic to cells, but what exactly does “glucotoxic” and “lipotoxic” mean?
An overabundance of glucose and free fatty acids leads to over-fueling of mitochondria. Since reactive oxygen species (ROS) are a byproduct of mitochondrial metabolism, increased amounts of ROS are formed when the cell experiences over-fueling.
Over-fueling will also trigger the rerouting of free fatty acids and glucose to alternative metabolic pathways, accumulating products that add to the cellular stress. Lastly, both ectopic fat and glycosylated proteins (advanced glycation products or AGEs) form, and further contribute to cellular stress. Organ function will decline. In susceptible individuals AGEs will fuel the progression of type-2 diabetes.
Increased cellular stress due to T2D and CVD sends the cell into a vicious cycle. Mitochondria function is at reduced capacity and ATP production declines. Damaged cells are more likely to undergo cell death, ER stress develops and cellular garbage increases.
The immune system tries to take care of cell fragments and cellular garbage. As a consequence systemic chronic inflammation increases.
Sounds familiar? These processes are very similar to cellular aging and the reason why metabolically unhealthy people experience the symptoms of aging earlier in life.
Genetic Predisposition and Lifestyle Choices Interact to Accelerate or Slow Aging
Inflammaging in healthy adults sounds more dramatic than it is. These processes are ongoing, but occur at a slow pace and healthy individuals leading a healthy lifestyle can be active and functional into high age.
In summary, as we age more proinflammatory cytokines are secreted due to normal environmental stressors (UV exposure, alcohol consumption, pollutants), increased production of neoplastic cells and cellular garbage. Cellular aging decreases the functionality of organs and increases the risk for age-related chronic diseases. If we look at centenarians it is possible to live a long, active life despite cellular aging processes.
Of course, our genetic predisposition will determine how sensitive we are to those stressors.
But, a positive, relaxed life in combination with a healthy lifestyle, including lots of physical activity seems to stem the tide. A plant-heavy diet supplying plenty of anti-inflammatory nutrients and antioxidants, and promoting a healthy gut microbiome reduces chronic inflammaging.
Cellular aging, combined with a genetic predisposition for chronic diseases and an unhealthy lifestyle, is a different story.
As discussed in the first section of the OER, most chronic diseases—such as T2D and CVD—and cancer develop when a genetic predisposition meets increased chronic systemic inflammation, often due to obesity and an unhealthy lifestyle. If chronic systemic inflammation is already ongoing, it adds to cellular aging and aggravates inflammaging. As a result, the aging process and development of chronic diseases are accelerated. Symptoms of aging such as physiological and cognitive decline start earlier.
On a side note, there is another aging factor that is less connected to nutrition: Immunosenescence, or the dysregulation of the immune system with high age.
The adaptive immune system produces what we call naïve immune cells that can recognize and memorize pathogens. Vaccination teaches naïve immune cells to recognize a pathogen in a safe way. Once the pathogen is encountered again, the adaptive immune defense is triggered.
The older people become, the less of those naïve cells they have. In old age, most of the naïve immune cells are already specialized to recognize specific pathogens. This not only makes old people more vulnerable to new pathogens, but also reduces the effectiveness of vaccinations. This explains, in part, why older people are so much more vulnerable to COVID-19, the flu, or other infectious diseases.
Back to Epigenetics: The Epigenome Changes Over a Lifetime
In the previous chapter we talked about chronological aging versus effective aging and attempts to determine the effective age by plotting chronic disease risk factors against the chronological age. The more effective way to determine effective or biological age are the epigenetic clocks.
Looking at the methylation pattern of the DNA—have a look at the epigenetics chapter as a refresher—scientists realized that the degree of methylation declines with age. Hypomethylation develops. In addition, the methylation pattern changes. While overall hypomethylation takes place, some CpG islands are hypermethylated. These changes alter how the epigenome activates or silences genes. Gene variations making somebody more vulnerable for a certain disease or altering a metabolic pathway become active.
The main reason for the age-related hypomethylation is that the enzymes that attaches methyl groups to the DNA of daughter cells during cell division (DNA Methyltransferases) become less active. Other factors promoting alterations to the DNA methylation pattern are adaptations to the environment and copy errors during cell division.
The interesting part is that the development of the DNA methylation pattern lines up with the chronological age of healthy people.
Scientists identified the methylation patterns of many people of various ages and used algorithms to design epigenetic clocks. Several epigenetic clocks are used in scientific aging research. This clocks are able to predict fairly well if a person experiences accelerated aging, the life expectancy and the risk for diseases.
We know that developing chronic diseases can speed up aging tremendously. So, the question is: how is aging sped up? One explanation is chronic systemic inflammation, but there is another explanation : changes to the epigenome. Metabolically unhealthy obesity and chronic diseases are connected to the acceleration of those epigenetic alterations which in turn profoundly alters the gene expression.
The goal for the future is to be able to identify people with a high risk for accelerated aging.
The good news is that the epigenome can be positively altered by a healthy lifestyle. It is thought that the key to changes of the DNA methylation pattern is lifestyle.
During the epigenetics unit, you learned that sufficient amounts of methyl tags are necessary to duplicate epigenetic marks during cell division, or to increase methylation to suppress genes.
A increased supply of acetyl tags alters histone modification. Exercise and calorie restriction reduce the supply of acetyl groups and therefore influence the epigenome beneficially.
A plant-heavy diet rich in anti-inflammatory nutrients and antioxidants promotes efficient regulatory enzymes reducing the progressing hypomethylation during the aging process. Soluble fiber and oligosaccharides form plant food also nudge the gut microbiome into a healthier composition. Some bacteria strains produce signaling molecules such as short chain fatty acids that influence the enzymes of the epigenome positively.
Maintenance of a healthy circadian rhythm—which can be disturbed during aging—seems to have an negative impact on the epigenome as well. In people with CVD, T2D or cognitive decline restricting the eating window to at least 11 – 12 hours if not less had also a positive impact on methylation patterns and metabolic health. Similar findings are available for periodical fasting.
The two explanations—inflammation and epigenetic changes due to lifestyle—are beginning to explain why we see such as diverse speed of the aging process in populations. Scientists are still working out why some individuals age faster than others, and why an increasingly larger group of centenarians seems to age extremely slowly. The goal of this aging research is to add as many healthy years to peoples’ lives as possible.
In summary: Physiological and cellular aging processes contribute to an increased risk for chronic diseases:
Physiology changes in healthy aging:
- Declining bone mineral density increases the risk for osteoporosis.
- Beta-cell function becomes less efficient, impacting blood glucose regulation negatively.
- Body composition becomes harder to maintain. Fat mass increases and as a consequence fat-free mass declines. Lean body mass is more dependent on exercise.
- Body fat shifts to central fat stores. Ectopic fat deposits increase and impact function of muscle and organs.
Cellular aging damages cell organelles and increases inflammation. Combined with epigenetic changes, the risk for age-related chronic diseases increases and the functionality of organs decrease:
- ROS (reactive oxidant species) damage mitochondria, leading to mitochondrial dysfunction. Less ATP is produced, leading to increased fatigue and cognitive decline.
- ER damage leads to misfolded protein accumulation; chronic systemic inflammation increases even in healthy individuals.
- Cellular waste removal is impaired, leading to increased systemic chronic inflammation.
- DNA damage increases risk for neoplastic cells.
- The DNA methylation pattern is altered.
Genetic predisposition in combination with lifestyle choice, accelerate or slow aging:
- Metabolically unhealthy obesity ↑
- Chronic diseases such as T2D and CVD ↑
- Genetic predisposition for chronic diseases ↑
- Declining DNA methylation and epigenome changes ↑
- Plant based diet ↓
- Healthy gut microbiome ↓
- Exercise ↓
The Key to Longevity? Dodging Chronic Diseases and Cognitive Decline
This closes the circle, bringing us back to the paradigm of the life cycle nutrition approach: The key to a long and healthy life is avoiding chronic diseases as long as genetically possible
As you have seen, the prevention of chronic diseases begins before birth and depends on mother’s behavior during pregnancy. This process continues after birth as a healthy metabolism is set by the feeding decisions parents make, and later the health behaviors learned from parents. Practicing a healthy lifestyle—or starting it during young adulthood—will reduce not only the risk for chronic diseases, but also slow the aging process as much as possible.
Overall, the contribution of our genes to aging is estimated to be 15-30%. The rest comes from our lifestyle decisions throughout life.
It should be clear that the speed of aging progression varies substantially. Therefore, rather than making assumptions and generalizing treatment for this age group, health care professionals should treat the heterogenous group of seniors on an individual basis.
Editors: Meryn Potts, Sydney Christensen
NUTR251 Contributors:
Spring 2020: Brittany Southall, Caleb Licking, Kennedie Engles, Allison Aden, Jenna Junker, Amelia Johnson, Hailie Slepicka, Melanie Nissen, Brittany Southall, Eli Havekost, Rose Davidson, Kyle Dawson, Mariel Sprakel, Julia Curtis, Colleen Sherman, Keeley Hagge, Rawan AL Jabri
Fall 2020: Morgan McCain
Underwater weighing, air displacement, bioelectrical impedance, skinfold measurements
Peptide hormone that that is produced in the pituitary gland and enables tissue and bone growth.
Insulin-like growth factor, a hormone, manages the effects of growth hormone. Together GH and IgF-1 manage growth of bones and tissues.
The final pathway for the oxidation of carbohydrates, fatty acids, and amino acids
Pathway breaking down fatty acids to acetyl-CoA, which is then oxidized in the citric acid cycle
Hypo indicates low
The prefix hyper indicates highly
Feedback/Errata
6 Responses to The Aging Process Explained